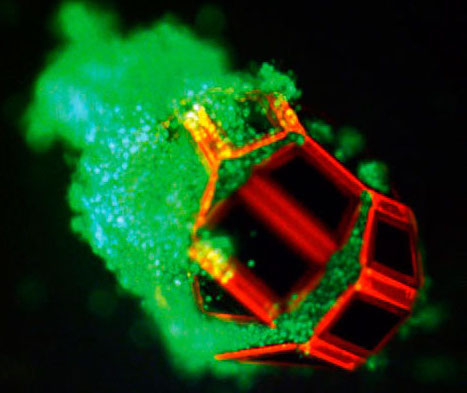
Biomedical micro/nano robotics
Imagine a tiny machine with moving parts that can sense its ambient, physiological, or marine environment and morph into different shapes to perform functional tasks like autonomously delivering drugs or excising tissue! To achieve this long-standing engineering dream, we have developed (a) sub-mm untethered thermobiochemically responsive biomedical robots powered by thin film microsprings and swelling gels, (b) introduced the concept of surgical surveillance using many small biopsy forceps as opposed to a large one, (c) and invented therapeutic grippers (theragrippers).
We developed the FIRST sub-mm sized, mechanized morphing structures such as grippers that can sense, fold or unfold in response to pH, temperature, proteases, glucose, L-glutamine, cell media, oxidative / reductive environments, and specific DNA sequences. IN addition, some designs can deliver significant forces during shape change. and were used to perform the FIRST ever biopsies and tissue sampling experiments in the gastrointestinal tract of live animals. Recently, theragrippers and microinjectors have been developed to deliver keterolac and insulin via the GI tract in vivo.
20. Pantula et al., Untethered unidirectionally crawling gels driven by an asymmetry in contact forces, Science Robotics (2022)
19. Ghosh et al., Autonomous untethered microinjectors for gastrointestinal delivery of insulin, ACS Nano (2022)
18. Jin et al., Untethered single cell grippers for active biopsy, Nano Letters (2020)
17. Ghosh et al, Active matter therapeutics, Nano Today (2020)
16. Xu et al., Soft three-dimensional robots with hard two-dimensional materials, ACS Nano (2019)
15. Ghosh et al., Stimuli-responsive soft untethered grippers for drug delivery and robotic surgery, Frontiers in Mechanical Engineering (2017)
14. Breger et al., Self-folding thermo-magnetically responsive soft-microgrippers, ACS Applied Materials and Interfaces (2015)
13. Yim et al, Biopsy using a magnetic capsule endoscope carrying, releasing and retrieving untethered microgrippers, IEEE Transactions on Biomedical Engineering (2014)
12. Malachowski, et al., Self-folding single cell grippers, Nano Letters(2014)
11. Malachowski,* Breger,* et al., Stimuli responsive theragrippers for chemomechanical controlled release, Angewandte Chemie International Edition (2014)
10. Gracias, Stimuli responsive self-folding using thin polymer films, Current Opinion in Chemical Engineering (2013)
9. Gultepe et al., Biopsy with thermally-responsive untethered microtools, Advanced Materials (2013)
8. Solovev et al., Rolled-up magnetic microdrillers: Towards remotely controlled minimally invasive surgery, Nanoscale (2013)
7. Solovev et al., Self-Propelled Nanotools, ACS Nano (2012)
6. Randhawa et al., Microchemomechanical Systems, Advanced Functional Materials(2011)
5. Bassik et al., Photolithographically Patterned Smart Hydrogel Based Bilayer Actuators, Polymer (2010)
4. Bassik et al., Enzymatically Triggered Actuation of Miniaturized Tools, JACS (2010)
3. Fernandes et al., Toward a miniaturized mechanical surgeon, Materials Today (2009)
2. Leong et al., Tetherless thermobiochemically actuated microgrippers, PNAS (2009)
1. Randhawa et al., Pick-and-place using chemically actuated microgrippers, JACS (2008)
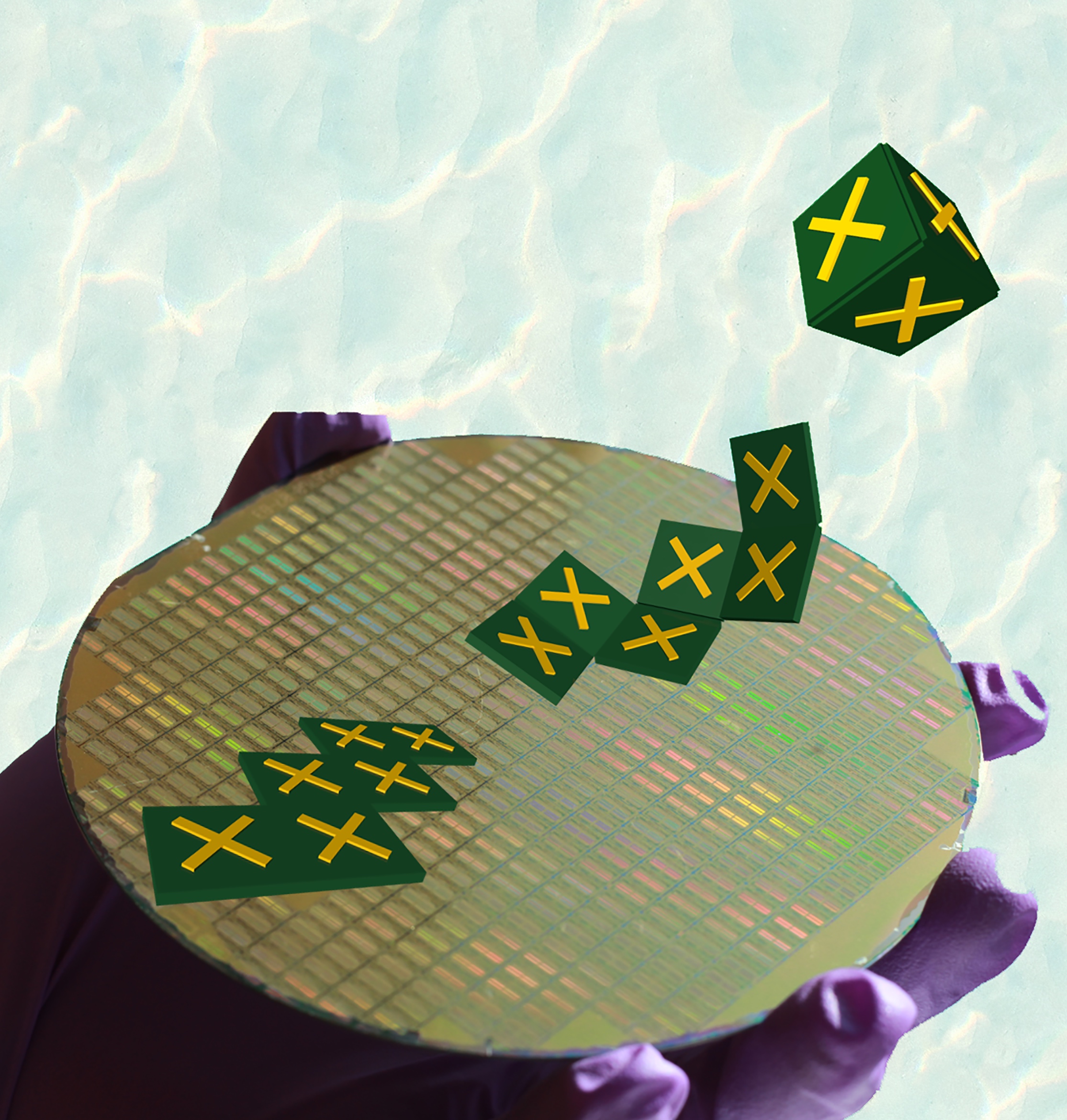
Origami/Kirigami MEMS and NEMS
We live in a 3D world, but conventional microchip (VLSI) patterning, which has revolutionized human life, is an inherently 2D process applicable only to a small set of materials. Imagine if we could leverage these established VLSI techniques and program forces to cause patterned 2D structures to fold up into 3D shapes spontaneously after release from the wafers. We would then have truly 3D devices and 3D metamaterials with micro and nanoscale dimensions manufactured in a highly parallel and cost-effective manner!
The Gracias lab has and continues to pioneer the concept of self-folding micro and nanoscale 3D metamaterials and devices. The laboratory has many FIRSTS, including self-folding of the smallest patterned 100 nm sized polyhedra, the concept of using trilayer hinges to make bidirectional self-folding microscale kirigami sheets, nanoscale origami for 3D optics, self-folding differentially UV crosslinked polymers.
17. Erol et al., Transformer hydrogels: A review, Advanced Materials Technologies (2019)
16. Kwok*, Wang,* et al., Nano-folded gold catalysts for electroreduction of carbon dioxide, Nano Letters (2019)
15. Xu et al., Reversible MoS2 origami with spatially resolved and reconfigurable photosensitivity, Nano Letters (2019)
14. Rogers et al., Origami MEMS and NEMS, MRS Bulletin (2016)
13. Yoon et al., Functional stimuli responsive hydrogel devices by self-folding, Smart Materials and Structures (2014)
12. Shenoy et al., Self-folding thin film materials: From nanopolyhedra to graphene origami, MRS Bulletin (2012)
11. Pandey et al., Algorithmic design of self-folding polyhedra, PNAS (2011)
10. Cho et al., Nanoscale Origami for 3D Optics, Small (2011)
9. Leong et al., Three dimensional fabrication at small size scales, Small (2010)
8. Cho et al., Curving nanostructures using extrinsic stress, Advanced Materials (2010)
7. Randhawa et al., Reversible actuation of microstructures by surface chemical modification of thin film bilayers, Advanced Materials (2010)
6. Bassik et al., Microassembly based on hands free origami with bidirectional curvature, Applied Physics Letters (2009)
5. Cho et al., Self-assembly of lithographically patterned nanoparticles, Nano Letters (2009)
4. Bassik et al., Patterning thin film mechanical properties to drive assembly of complex 3D structures, Advanced Materials (2008)
3. Leong et al., Thin film stress driven self-folding of microstructured containers, Small (2008).
2. Leong et al., Surface tension driven self-folding polyhedra, Langmuir (2007)
1. Gracias et al., Fabrication of micrometer-scale, patterned polyhedra by self-assembly, Advanced Materials (2002)
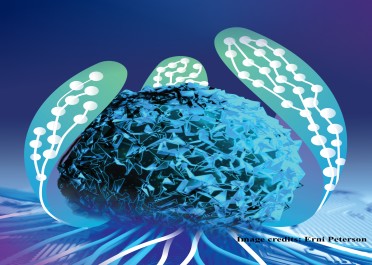
Bio-origami, Biomimetics and 3D microphysiological systems
The human body is incredibly well patterned on the mm, micro, and nanoscale in complex 3D, curved, and folded architectures. Humans are unable to replicate these complex micropatterned environments with biologically relevant materials. The Gracias laboratory has and continues to pioneer morphogenesis inspired approaches of self-folding biomedical devices including those for drug delivery, tissue engineering and surgery. The laboratory has many FIRSTS including introducing the concept of “bio-origami” hydrogels for tissue engineering and “self-folding microfluidics, and capsules”. We invented 3D MEA shells for brain organoids and “polymerization gels”, a new type of active gel that can be programmed using DNA sequences.
17. Shi et al, Programming gel automata shapes using DNA instructions ,Nature Communications (2024)
16. Kwok,* Zuo,* Choi* et al, Toward single cell tattoos: Biotransfer printing of lithographic gold nanopatterns on live cells ,Nano Letters (2023)
15. Huang et al, Shell microelectrode arrays (MEAs) for brain organoids, Science Advances (2022)
14. Jin,*Bhatta,*Pagaduan,*Chen,* et al., Biomimetic human small muscular pulmonary arteries, Science Advances (2020)
13. Shi et al., Multicomponent DNA polymerization motor gels, Small (2020)
12. Cools et al, A micropatterned multielectrode shell for 3D spatiotemporal recording from live cells, Advanced Science (2018)
11. Cangialosi,* Yoon,* et al. DNA sequence-directed shape change of photopatterrned hydrogels via high-degree swelling, Science (2017)
10. Jin,* Li* et al., Mechanical Trap Surface Enhanced Raman Spectroscopy (MTSERS) for 3D surface molecular imaging of single live cells, Angewandte Chemie (2017)
9. Xi et al., Molecular insights into division of single human cancer cells in on-chip transparent microtubes, ACS Nano (2016)
8. Mannoor et al., 3D printed bionic ears, Nano Letters (2013)
7. Jamal et al., Bio-origami hydrogel scaffolds composed of photocrosslinked PEG bilayers, Advanced Healthcare Materials (2013)
6. Fernandes et al., Self-folding polymeric containers for encapsulation and delivery of drugs, Advanced Drug Delivery Reviews (2012)
5. Randall et al., Self-folding materials and devices for biomedical applications, Trends in Biotechnology (2012)
4. Jamal et al., Differentially photo-crosslinked polymers enable self-assembling microfluidics, Nature Communications (2011)
3. Azam et al, Self-folding micropatterned polymeric containers, Biomedical Microdevices (2011)
2. Jamal et al, Directed growth of fibroblasts into three dimensional micropatterned geometries via self-assembling scaffolds, Biomaterials (2010)
1. Randall et al., 3D lithographically fabricated nanoliter containers for drug delivery, Advanced Drug Delivery Reviews (2007)
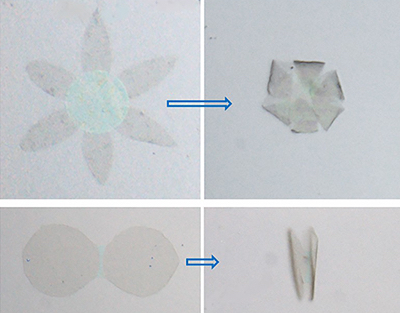
Folding atomically thin films
Imagine taking atomically thin paper and folding it into 3D shapes. We might then be able to make skin-like wearables or robots. To enable this engineering dream, we have pioneered the self-folding and origami engineering of atomically thin films like graphene and MoS2. Of note, we invented thermally responsive “self-folding graphene”.
3. Xu et al., Self-folding hybrid graphene skin for 3D biosensing, Nano Letters (2019)
2. Xu et al., Ultrathin shape change smart materials, Accounts of Chemical Research (2018)
1. Xu et al., Ultrathin thermoresponsive self-folding 3D graphene, Science Advances (2017)
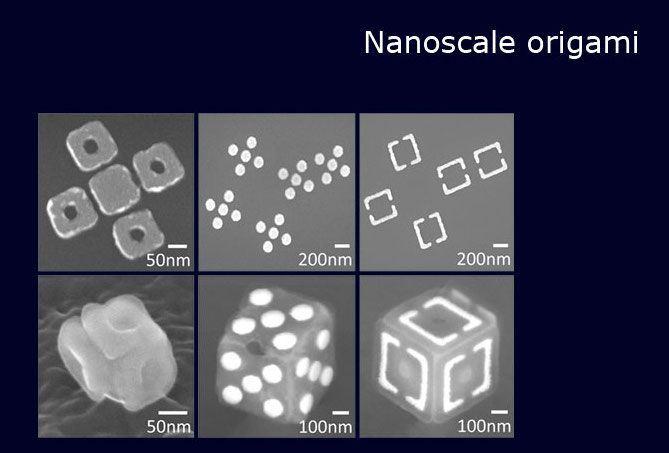
Self-assembly by programmed folding and aggregation
Nature fabricates complex structures like living cells, mountains, and galaxies by hierarchical self-organization; these fabrication paradigms are largely unused in human engineering. Imagine, for example, if we could self-organize a computer from a collection of transistors. To realize this engineering dream, we have pioneered the self-assembly of arrayed, 3D, integrated devices by aggregation and folding. Some of our pioneering FIRSTS include “self-assembling circuits with serial and parallel connectivity”, “self-folding of polyhedral sensors and 2D chips”, and “self-aggregating THz metamaterials”
4. Pandey et al., Algorithmic design of self-folding polyhedra, PNAS (2011)
3. Jacobs et al., Fabrication of a cylindrical display by patterned assembly, Science (2002)
2. Boncheva et al., Biomimetic self-assembly of a functional asymmetrical electronic device, PNAS (2002)
1. Gracias et al., Forming electrical networks in three dimensions by self-assembly, Science (2000)
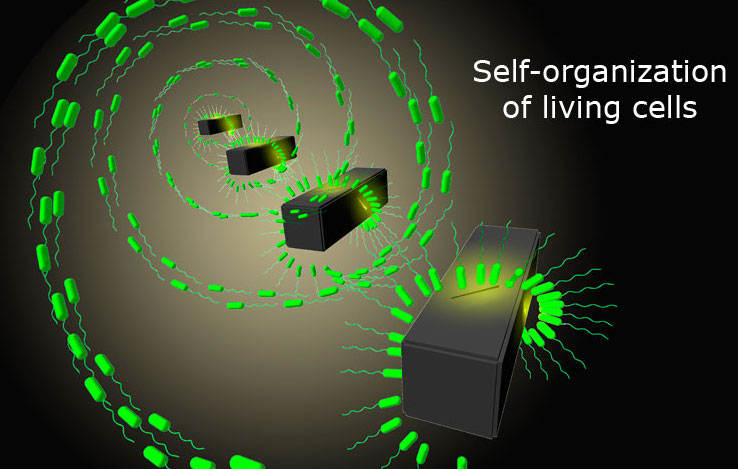
Spatio-temporally controlled chemistry
In laboratories, we perform chemical reactions by mixing reactants in flasks and beakers, quite unlike what happens in and around living cells. In cells, reactants are encapsulated in organelles and chemistry is controlled precisely in space and time through programmed reaction-diffusion. We have pioneered “spatiotemporally controlled chemistry” including the first demonstration of spatially controlled microscale chemistry with precisely patterned porosity on self-folded microcapsules. We also invented and patented a”chemical display” which can generate animations not by electronics and optical pixels but by spatiotemporally programmed diffusion of dyes or fluorescent chemicals. This display does not need any batteries or external power.
3. Kalinin,* Pandey,* et al., A chemical display: Generating animations by controlled diffusion from porous voxels, Advanced Functional Materials (2016)
2. Ye et al., Remote radio frequency controlled nanoliter chemistry and chemical delivery on substrates, Angewandte Chemie International Edition (2007)
1. Leong et al., Spatially controlled chemistry using remotely guided nanoliter scale containers, JACS (2006)
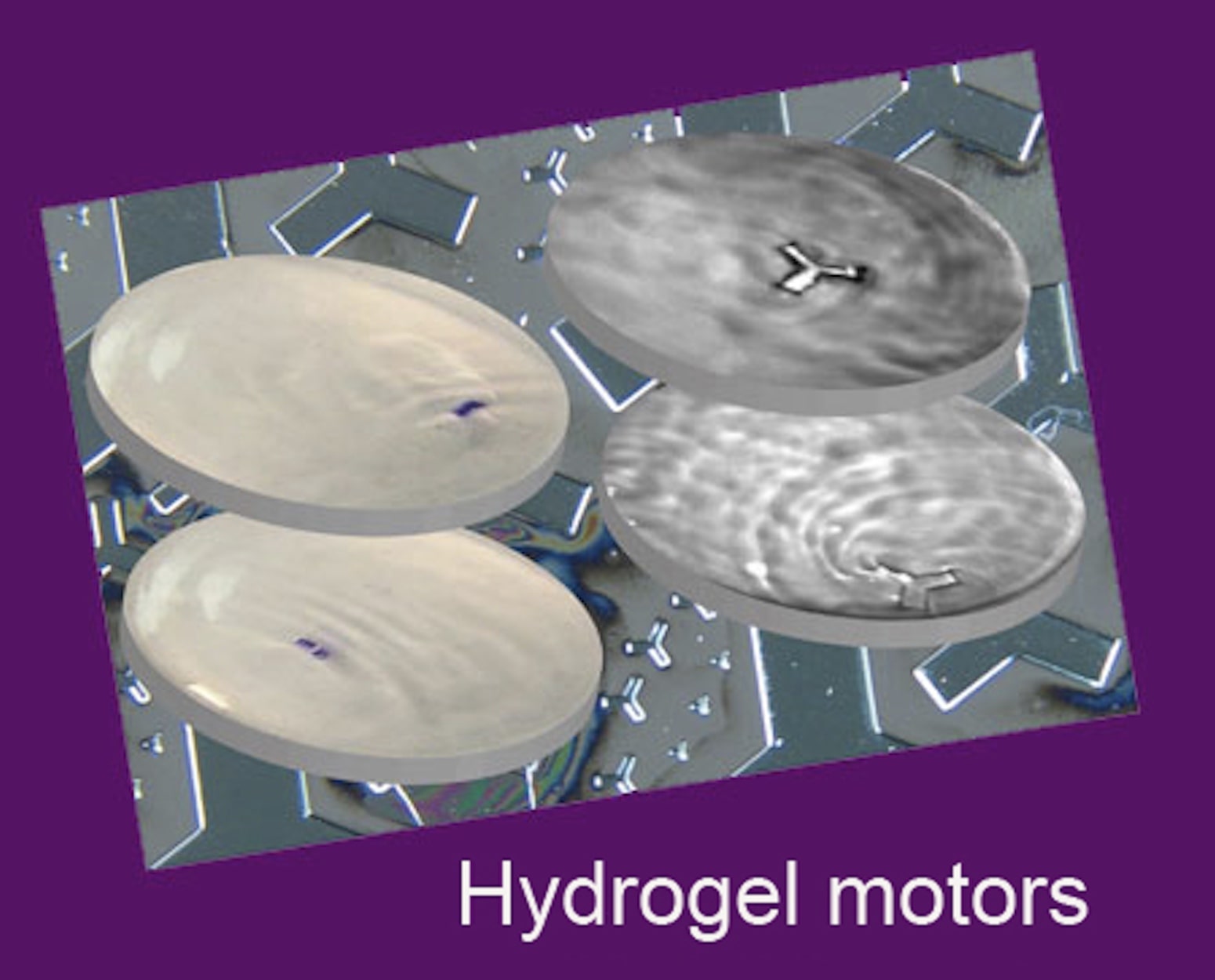
Surface science, spectroscopy, and interesting phenomena
Our students are problem solvers. We have addressed many societal problems that need urgent scientific and technological solutions.
9. Paria*, Kwok* et al, Label-free spectroscopic SARS-CoV-2 detection on versatile nanoimprinted substrates, Nano Letters (2022)
8. Chowdhury et al., Substrate directed synthesis of MoS2 nanocrystals with tunable dimensionality and optical properties, Nature Nanotechnology (2019)
7. Bassik et al., Solvent driven motion of lithographically fabricated gels, Langmuir (2008)
6. Ye et al., Probing organic field effect transistors in-situ during operation using SFG, JACS (2006)
5. Ye et al., Kinetics of ultraviolet and plasma surface modification of poly(dimethylsiloxane) probed by sum frequency vibrational spectroscopy, Langmuir (2006)
4. Mayer et al., Micropatterned agarose gels for stamping arrays of proteins and gradients of proteins, Proteomics (2004)
3. Gracias et al., Molecular characterization of polymer and polymer blend surfaces. Combined sum frequency generation surface vibrational spectroscopy and scanning force microscopy studies, Accounts of Chemical Research (1999)
2. Yang et al., Lithographic fabrication of model systems in heterogeneous catalysis and surface science studies, Langmuir (1999)
1. Gracias et al., Continuum force microscopy study of the elastic modulus, hardness and friction of polyethylene and polypropylene surfaces, Macromolecules (1998)